10/1/2021
Producing High-Quality Liners With Root-Zone Heating
Annika Kohler & Roberto Lopez
High-quality liners are generally compact, have thick stems, small and dark green leaves, and an adequate root and shoot mass for successful shipping and transplanting. Additionally, liners should be free of buds and flowers. However, propagation of unrooted cuttings in northern latitudes largely takes place during late winter and early spring to ensure a consistent supply of young plants for the spring bedding plant season.
Due to the seasonality of young plant production, growers can face high energy costs for heating and supplemental lighting to provide optimal temperatures and daily light integrals (DLIs) for rooting. However, one way to mitigate heating costs is to propagate cold-tolerant crops that have relatively low base temperatures (can still develop at temperatures <39F [4C]) rather than cold-intermediate and cold-sensitive crops (Figure 1 and Table 1).
Table. 1. Estimated base temperature (Tb) of cold-tolerant floriculture crops from research conducted at Michigan State University.
Cold Tolerant (Low Tb) <39F
Alyssum
Calibrach
Campanula
Dianthus
Diascia
America marigold
French marigold
Nemesia
Nepe
Osteospermum
Petunia (Bravo, Dreams and Easy Wave)
Phlox
Snapdragon (Liberty Classic and Montego)
Rosemary
Stock
Viola
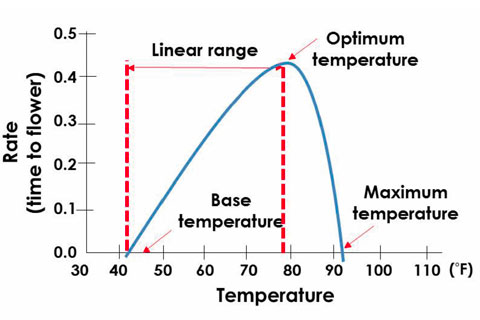
The experiment
Unrooted cuttings of Calibrachoa Callie Coral (Calibrachoa ×hybrida), Campanula Birch Hybrid (Campanula portenschlagiana), Nemesia Aromatica Royal Blue (Nemesia fruticans), Nepeta Junior Walker (Nepeta ×faassenii), Osteospermum Voltage Yellow (Osteospermum ecklonis), Petunia Sanguna Patio Blue (Petunia ×hybrida), Phlox Glamour Girl (Phlox paniculata), and Rosemary Arp (Rosmarinus officinalis) were received from commercial suppliers and stuck into 72-cell trays filled with a 1:1 volume-to-volume of a commercial soilless substrate (70% peat moss, 21% perlite and 9% vermiculite) and medium perlite.
Figure 1. An example of the effect of temperature on developmental rates, such as time to flower. The base temperature is the species-specific temperature at which plant development stops, the optimum temperature is the temperature where plant development is most rapid and the maximum temperature is the temperature where development stops.
For callusing, trays were placed in a glass-glazed greenhouse compartment in the Plant Science Greenhouse Complex at Michigan State University, which had an air temperature and bench-top root-zone heating set point of 70F (21C) and 75F (24C), respectively. High-pressure sodium lamps provided 62 ± 6 µmol•m–2•s–1 of supplemental light when the outdoor light intensity was below 440 µmol•m–2•s–1. A ~50% shade cloth was placed over the benches to achieve a DLI of 5 mol•m–2•d–for 6 days.
After callusing, trays were placed among six different temperature treatments, which included an air temperature of 60 or 70F (16 or 21C) and three root-zone heating temperatures of 70, 75 or 80F (21, 24 or 27C). Supplemental lighting was utilized to provide a DLI of 11 to 12 mol•m–2•d–1 for rooting. Over-head mist providing 60 ppm N was used intermittently based on light intensity, reduced every few days and turned off one week prior to data collection to allow liners to harden off.
From then on, plants were hand-irrigated with 60 ppm N. A vapor pressure deficit of 0.3 kPa was maintained using steam injection. After 14 days, 10 liners per genus and treatment were harvested and the soilless substrate was gently rinsed from the roots, and roots and shoots were separated and dried to measure the root and shoot dry mass.
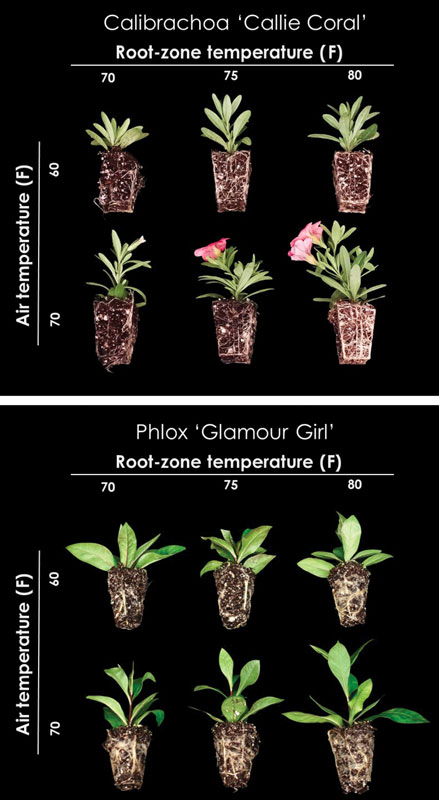
What did we learn?
Increasing the air and/or root-zone temperature resulted in increased stem elongation of calibrachoa (Figure 2), nemesia, nepeta and rosemary. For instance, at an air temperature of 70F, increasing the root-zone temperature from 70 to 80F resulted in the stem length of calibrachoa, nemesia and rosemary, increasing by 16%, 13% and 14%, respectively.
Furthermore, air and root-zone temperature interacted to influence the stem length of campanula, nepeta, petunia, phlox (Figure 3) and rosemary. For example, as we increased the air temperature from 60 to 70F, the stem length of nepeta increased by 37% to 49% as root-zone temperature increased from 70 to 80F, respectively. Neither air nor root-zone temperature influenced stem caliper of any genera studied except for petunia. This isn’t surprising, as DLI generally influences stem caliper to a greater extent than temperature.
Figure 2. Rooted cuttings of Calibrachoa Callie Coral (Calibrachoa ×hybrida) after 14 days of root-zone and air temperature treatments during propagation.
From our previous research, we’ve determined that a DLI of 10 to 12 mol•m–2•d–1 should be maintained to produce an abundance of roots and compact, well-branched shoots (quantified as root and shoot dry mass) and ultimately reduce rooting time. Here we determined that root-zone temperature influenced the root dry mass of calibrachoa, nemesia and rosemary, whereas air temperature influenced the root dry mass of campanula, nepeta and rosemary.
For instance, the root dry mass of calibrachoa was greatest when the root-zone temperature was 70F, while nemesia and rosemary had greater root dry masses at a root-zone temperature of 75F. At a root-zone temperature of 75F, the root dry mass of rosemary was 26% greater under an air temperature of 60F compared to 70F.
Figure 3. Rooted cuttings of Phlox Glamour Girl (Phlox paniculata) after 14 days of root-zone and air temperature treatments during propagation.
Additionally, the interaction of air and root-zone temperature influenced the root dry mass of petunia and osteospermum. For example, as the root-zone temperature increased from 70 to 80F, the root dry mass of petunia decreased by 27% at an air temperature of 70F. Furthermore, as the air temperature increased from 60 to 70F, the root dry mass was reduced by 24% at a root-zone temperature of 80F.
The shoot dry mass of calibrachoa, nemesia and nepeta increased with increasing air temperature, while increasing the root-zone temperature increased the shoot dry mass of nepeta, but variably influenced nemesia, petunia and rosemary. As root-zone temperature increased from 70 to 80F, the shoot dry mass of nepeta was 23% and 28% greater at an air temperature of 60 and 70F, respectively. The interaction of air and root-zone temperature influenced the shoot dry mass of calibrachoa, petunia and phlox. As root-zone temperature increased from 75 to 80F, the shoot dry mass of calibrachoa and petunia was reduced at an air temperature of 60F.
What can we conclude given that there are differences between the genera?
We found that either air or root-zone temperature influenced stem elongation of six of the eight genera we investigated. Therefore, producing cold-tolerant liners at cooler air temperatures can control excessive stem elongation, reduce premature flowering and potentially create a higher-quality liner without the use of PGRs. Although we’ve determined that it’s possible to propagate cold-tolerant crops at cooler-than-normal propagation air temperatures, it’s best to provide floor or bench top root-zone heating to reduce any developmental delays, which could cause slow root or shoot growth.
However, root-zone temperatures >75F in combination with warm air temperatures should be avoided, as they can negatively affect root development and promote excessive shoot growth. A root-zone temperature of 70F should also be avoided if you’re propagating at cooler air temperatures. Therefore, cold-tolerant crops can be propagated without sacrificing quality at an air temperature of 60F and a root-zone temperature of at least 75F. GT
Acknowledgements: The authors gratefully acknowledge Michigan State University’s Project GREEEN, The Western Michigan Greenhouse Growers Association, The Metro Detroit Flower Growers Association and the Fred C. Gloeckner Foundation for funding, as well as horticultural companies for providing support of Michigan State University’s floriculture research. We would also like to thank Nate DuRussel for greenhouse assistance and data collection, as well as Ball Horticultural Company, Syngenta Flowers and Dümmen Orange for donating plant material. This research was supported by the USDA National Institute of Food and Agriculture, Hatch project MICL02472.
Annika Kohler is a Research Technician and Roberto Lopez is an Associate Professor and Controlled Environment/Floriculture Extension Specialist in the Department of Horticulture at Michigan State University.